Nonlinear Distortion Compensation Technology for Achieving Large-capacity, Long-distance Optical Networks
Release date: August 5, 2020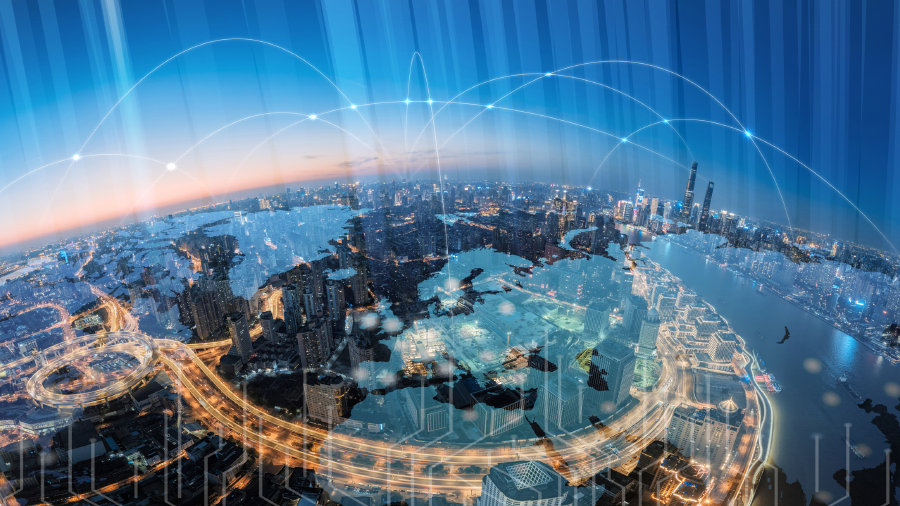
The optical network accommodating massive amounts of data traffic has increased its capacity dramatically by ten times over the last five years. From here on, however, it will be necessary to achieve even larger capacities in the 400 Gbps—1 Tbps per wavelength class beyond today’s mainstream optical transmission capacity of 100 Gbps to accommodate ever-increasing volumes of traffic. To achieve this, it will be necessary to overcome waveform distortion (nonlinear distortion) in the optical signal caused by nonlinear optical effects in the optical fiber transmission, which is a remaining limiting factor that hinders increases in capacity. A number of relatively simple proposals for mitigating such nonlinear distortion have been made at academic societies, but the circuit scale of these proposals has been extremely large preventing them from being realistic solutions. We have overcome this problem by proposing a nonlinear distortion compensation method that greatly reduces the computational complexity of nonlinear distortion compensation with respect to multilevel and polarization multiplexing in current optical transmission. Using this technology, we have achieved a practical optical transceiver equipped with nonlinear distortion compensation as a world’s first.
In this article, we describe nonlinear distortion compensation technology for large-capacity optical transceivers.
- 1. Introduction
- 2. Prior technology
- 3. Newly developed nonlinear distortion compensation technology
- 4. Evaluation
- 5. Conclusion
1. Introduction
The amount of data traffic flowing through the Internet has been increasing at an annual rate of 1.2 or greater thanks to the proliferation of smartphones, cloud services, video delivery services, etc. [1]. Going forward, we can expect an explosive increase in traffic due to the appearance of new services and the launching of the fifth-generation mobile communications system (5G). The optical network—the core network supporting the Internet—has increased its capacity by ten times over the last five years, but achieving even higher capacities will be absolutely necessary to deal with this explosive increase in traffic.
The current commercial optical network commonly supports an optical transmission capacity of 100 Gbps per wavelength. However, to continue to accommodate increasing volumes of data traffic in the years to come, it will be necessary to achieve even higher capacities such as 400 Gbps—1 Tbps and longer optical transmission distances. This, though, will require measures to deal with waveform distortion (nonlinear distortion) in the optical signal caused by nonlinear optical effects in the optical fiber transmission, which is a remaining limiting factor hindering increases in capacity.
In general, it is essential that the optical signal-to-noise ratio (OSNR) be improved to achieve an optical communications system capable of large-capacity, long-distance transmission. However, there are physical limitations to reducing optical noise power at optical amplifiers, so it is desirable to improve OSNR by increasing optical signal power. On the other hand, increasing optical signal power in optical fiber transmission results in significant distortion of the signal waveform due to a nonlinear effect (optical Kerr effect) that occurs in glass as a property of optical fiber[2]. One technology that has been proposed for dealing with this distortion is compensation of nonlinear distortion through digital signal processing (Figure 1) [3,4]. With this technology, compensation of nonlinear distortion generated by high optical signal power can be expected to improve OSNR thereby improving signal quality. However, there is currently no method for achieving both this effect and a circuit of feasible scale, which has become an issue hindering practical implementation.
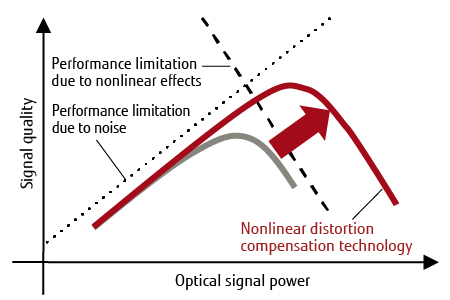
Figure 1 Effect of nonlinear distortion compensation technology.
In response to this issue, we developed a nonlinear-distortion-compensation digital signal processing circuit that significantly reduces the computational complexity of nonlinear distortion compensation and operates with a realistic circuit scale and level of power consumption. We accomplished this by splitting the nonlinear waveform distortion factor in optical fiber into multiple detailed distortion factors and by introducing a new approximate nonlinear distortion analysis formula. Furthermore, by mounting this nonlinear-distortion-compensation circuit in an optical transceiver, we succeeded in easing the performance limitations originating in nonlinear distortion caused by an increase in optical signal power thereby increasing the capacity and distance of transmissions [5,6].
In this article, we describe this nonlinear distortion compensation technology for achieving large-capacity, long-distance optical transmission.
We note here that we, the authors, received a Prize for Science and Technology (Development Category) in the 2020 Commendation for Science and Technology by the Minister of Education, Culture, Sports, Science and Technology for “Development of Optical Nonlinear Distortion Compensation Technology for Large-capacity Optical Transceivers” [7].
2. Prior technology
Nonlinear distortion forms a harmonious whole with linear distortion (known as chromatic dispersion in optical fiber) that occurs simultaneously within the optical fiber resulting in complex inter-symbol interference. Even before 2008 when study of the proposed technology began, a number of compensation techniques for this complex inter-symbol interference had been discussed, but they all required exceedingly complicated circuits that formed a high barrier to practical use.
One of the most widely known of these prior techniques is the digital backpropagation method that compensates for nonlinear distortion by reversely propagating the optical signal within the optical fiber in a virtual manner through digital signal processing (Figure 2). The backpropagation method adopts the ideas behind the split-step Fourier method, which is a numerical analysis technique for basic equations (nonlinear Schrödinger equation and Manakov equation) describing optical propagation within an optical fiber. This technique expresses the propagation of an optical signal within an optical fiber by repeated calculation of linear and nonlinear effects. On the optical receiver side, this technique is used to compensate for linear and nonlinear distortion throughout the optical fiber transmission path by applying it repeatedly to each linear equalizer (LE) circuit and nonlinear equalizer (NLE) circuit having characteristics opposite those of the optical fiber transmission path.
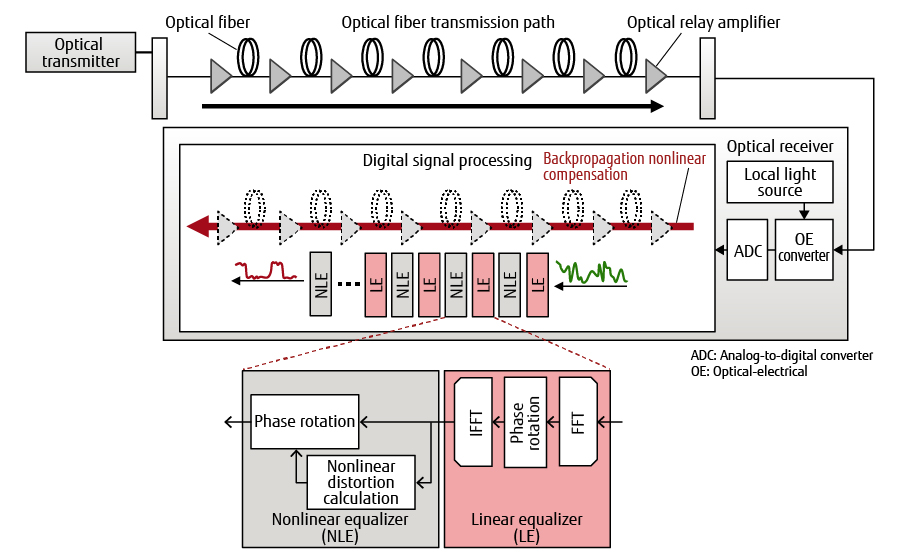
Figure 2 Backpropagation-type nonlinear distortion compensation technology.
In backpropagation, the circuit combining an LE and NLE pair is treated as a basic block, and increasing the number of blocks (number of stages of repeated calculations) can enhance the performance of distortion compensation including that for nonlinear distortion [8]. However, this basic block includes a large-scale FFT/IFFT [9] circuit, so connecting multiple blocks in many stages results in a massively large circuit overall. As a result, this was a technology that could not be easily implemented considering the circuit scale and power consumption that would be required of actual optical transceivers.
3. Newly developed nonlinear distortion compensation technology
In response to the problems described in the previous section, we developed new nonlinear distortion compensation technology capable of greatly reducing circuit scale. This technology splits the distortion caused by nonlinear optical effects in optical fiber into intra-channel nonlinear distortion arising from optical signals within the signal band and inter-channel nonlinear distortion arising from neighboring optical signals outside the signal band, and efficiently compensates for each type of distortion by separate compensation circuits. For intra-channel nonlinear distortion compensation, we introduced a new approximate nonlinear distortion analysis formula to significantly decrease computational complexity and simplify the circuit reducing it to 1/10 its previous size. Next, in inter-channel nonlinear distortion compensation, we focused on nonlinear polarization crosstalk, which is the inter-channel nonlinear distortion unique to polarization multiplexing transmission systems used as standard in optical transmission above 100 Gbps, and established a technique that could efficiently compensate for this distortion. With these techniques, we achieved high nonlinear distortion compensation performance as well as a significant reduction in circuit scale and succeeded in implementing this technology for practical use as a world’s first.
This section describes two key elemental technologies making up this nonlinear distortion compensation technology.
3.1 Intra-channel nonlinear compensation technology for reducing computational complexity
Here, with the aim of efficiently compensating for intra-channel nonlinear distortion, we focused on the basic equation of polarization multiplexing transmission (Manakov equation) and proposed an approximate nonlinear distortion analysis formula based on nonlinear perturbation analysis that greatly reduces computational complexity.
In the prior compensation method, 10 stages or more of the basic block (LE + NLE) were required to emulate a long-distance transmission path consisting of multiple optical fibers and optical amplifiers as shown in Figure 3. In contrast, the algorithm shown in Figure 4 based on perturbation analysis takes into account the interaction between pulses due to pulse broadening caused by linear distortion in optical fiber and the interaction between polarized signals. This improves the performance of the nonlinear distortion compensation circuit and greatly minimizes any increase in circuit scale (Figure 3). In this way, the proposed nonlinear distortion compensation method can achieve distortion compensation performance equivalent to that of prior technology with fewer basic-block stages smaller by an order of magnitude.
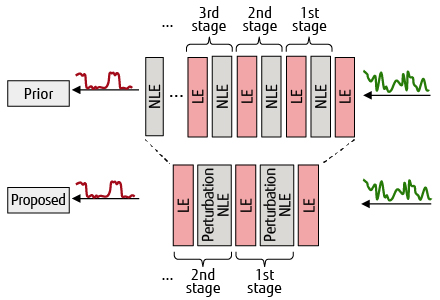
Figure 3 Reduction of computational complexity by a perturbation-type nonlinear distortion compensation method.
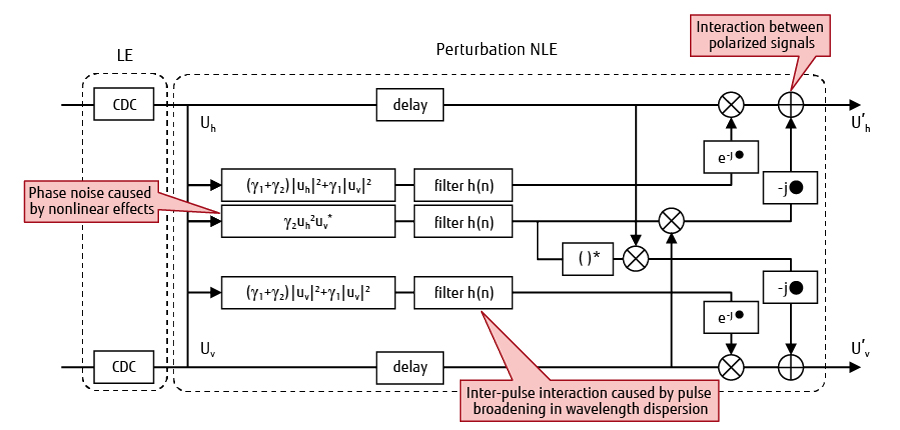
Figure 4 Nonlinear distortion compensation algorithm using a perturbation analysis technique.
3.2 Inter-channel nonlinear compensation technology
Wavelength multiplexing transmission technology that transmits multiple channels via multiple wavelengths has been used widely in optical fiber transmission. However, the transmission performance of a wavelength multiplexing transmission system is limited by nonlinear distortion caused by neighboring wavelength channels. In this research, we proposed a nonlinear polarization crosstalk canceller that can effectively compensate for nonlinear polarization crosstalk—one type of inter-channel nonlinear distortion—with a small-scale digital circuit. In this regard, nonlinear polarization crosstalk frequently occurs when introducing optical signals of even greater capacity in stages in an existing system in which intensity-modulated signals of 10 Gbps have already been introduced. Using optical transceivers equipped with the proposed nonlinear polarization crosstalk canceller suppresses nonlinear distortion that occurs when introducing large-capacity optical signals in existing optical fiber networks and therefore facilitates capacity increases in optical networks in an economical manner.
4. Evaluation
In this section, we describe a performance evaluation of the developed technology.
Figure 5 shows the results of evaluating the performance of a 100 Gbps optical transmission system using an LSI equipped with the developed nonlinear compensation circuit. Using the developed nonlinear distortion compensation technology, we achieved an OSNR approximately 3 dB lower for the same bit error rate (BER), which indicates the possibility of extending transmission distance by up to two times. On comparing the waveforms of received signals, it can be seen that the developed technology (nonlinear distortion compensation technology) decreased the scattering of constellation points and improved the quality of the demodulated waveform. This evaluation used 100 Gbps optical signals, but applying the proposed technology to optical signal transmission in the 400 Gbps—1 Tbps class should enable even higher levels of large-capacity, long-distance transmission.
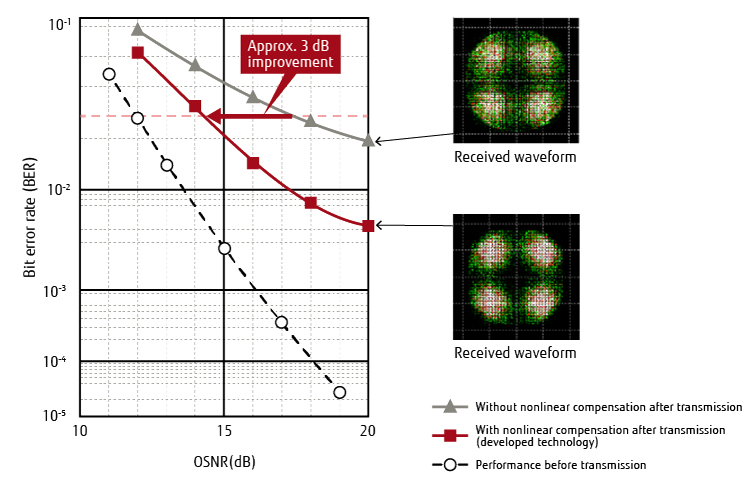
Figure 5 Performance evaluation results.
5. Conclusion
This article described nonlinear distortion compensation technology for large-capacity optical transceivers.
In 2008, the study of nonlinear distortion compensation originated in the academic field, but the massively large circuit proposed left little hope for practical implementation. Against this background, we went on to present many papers including a number of invited talks at domestic and international conferences in the field of optical communications and to disseminate research results and promote technologies related to nonlinear distortion compensation. Through these efforts, we helped to form a major research field involving universities and even overseas competitors and accelerate technology development in this area. Furthermore, through a trial-and-error process based on both academic and practical-oriented research, we achieved a significant reduction in circuit scale and the world’s first practical implementation of nonlinear distortion compensation technology after a period of nine years.
Going forward, we aim to drive the ongoing evolution of ultra-large-capacity, long-distance optical communication networks through further advances in this technology. We can envision these networks as a foundation for an advanced information society covering the entire world.
This work was partly supported by “The Research and Development Project for the Ultra-high Speed and Green Photonic Networks” of the Ministry of Internal Affairs and Communications, Japan and by the National Institute of Information and Communications Technology (NICT), Japan.
All company and product names mentioned herein are trademarks and registered trademarks of their respective owners.
References and Notes
- G. P. Agrawal: Nonlinear Fiber Optics, Fourth Edition, Academic Press, October 2006.Back to Body
- G. Goldfarb et al.: Demonstration of fiber impairment compensation using split-step infinite-impulse-response filtering method. Electron. Lett., Vol. 44, No. 13, pp. 814–816, June 2008.Back to Body
- T. Hoshida et al.: Digital nonlinear compensation for spectrally efficient superchannel transmission at 400Gbit/s and beyond.European Conference on Optical Communication and Exhibition on Optical Communication, paper We.3.3.1, September 2014.Back to Body
- L. Li et al.: Nonlinear Polarization Crosstalk Canceller for Dual-Polarization Digital Coherent Receivers.The Optical Fiber Communication Conference and Exhibition/National Fiber Optic Engineers Conference (OFC/NFOEC), OWE3, March 2010.Back to Body
- W. Yan et al.: Low Complexity Digital Perturbation Back-propagation. European Conference and Exhibition on Optical Communication, paper Tu3. A.2, September 2011.Back to Body
- E. Ip et al.: Compensation of Dispersion and Nonlinear Impairments Using Digital Backpropagation. J. Lightwave Technol., Vol. 26, No. 20, pp. 3416–3425, Oct. 2008.Back to Body
- Abbreviations for “fast Fourier transform” and “inverse fast Fourier transform.” These are algorithms for performing discrete Fourier transforms that convert the signal from the time domain to the frequency domain or vice versa at high speed on a computer.Back to Body
About the Authors
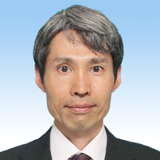
Dr. Hoshida is engaged in the development of ultra-high-speed, long-distance optical transmission system technology.
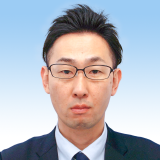
Mr. Nakashima is engaged in the development of digital signal processing technology for ultra-high-speed, long-distance optical transmission.
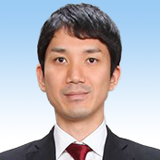
Dr. Oda is engaged in the development of high-efficiency-transmission and operation-automation technologies for ultra-high-speed, long-distance optical transmission.
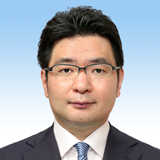
Dr. Tanimura is engaged in the development of ultra-high-speed, long-distance optical transmission, transmission-oriented digital signal processing, and network state inference and operation automation using machine learning and deep learning.